The Irradiance Optimation for Growing Spirulina fusiformis: Biomass, Phycocyanin, and Protein Production
DOI:
https://doi.org/10.51264/inajl.v2i1.7Keywords:
cyanobacterium microalgae, photobioreactor, phycocyanin, irradiance, Spirulina fusiformisAbstract
The optimization of cyanobacterium microalgae cultivation technology to provide the need for food or feedstocks has recently attracted many investigators. An optimum operation on microalgae cultivation is important to reduce the excessive workload on the aquatic environment. Therefore, this study describes how the varied irradiance (2000 lux, 4000 lux, 6000 lux, 8000 lux, and 10,000 lux) treatments on a bubble column photobioreactor system affected biomass production, phycocyanin, and protein from cyanobacterium Spirulina fusiformis. The objective of this study was to obtain the optimum irradiance for producing maximum biomass, phycocyanin, and protein simultaneously. The results demonstrated some findings those were: 1) irradiance 10,000 lux made doubling time of growth earliest (only 24 hours) while 2,000 lux doubled within five days later; 2) light response curve showed that the increase of biomass concentration was linear with the increasing of irradiance; 3) a predictive model (Response Surface Method) proof that the most optimum quantity of the biomass (0.58 ±0.035 gL-1 dry weight), chlorophyll-a (0.090 ±0.023 % dry weight), and phycocyanin (2.44 ±0.00 gL-1 dry weight) were obtained on 10,000 lux, while protein contents of 79.18±5.47 % dry weight attained on the irradiance of 6000 lux. The maximum productivity of the biomass, chlorophyll-a, phycocyanin, and protein was ~Pbiomass of 24.95 mgL-1day-1; Pchl-a of 2.25E-02 mgL-1day-1; Pphycocyanin of 1.88E-02 mgL-1day-1; and Pprotein of 17.56 mgL-1day-1. Enhancement of irradiance up to 5 folds lead to the increasing of biomass chlorophyll-a, phycocyanin, and protein productivity, attained to 1.7, 5.01, 4.13, and 2.81 folds, respectively. The irradiance had a significant influence on the production of the metabolites; therefore, the irradiance must be optimized.
References
Akashi T, Okajima M, Kaneko T. 2008. Extraction of chromo proteins from Aphanothece sacrum and their applications to optically functional materials. Journal of Biotechnology, 136, Supplement, S450. https://doi.org/http://dx.doi.org/10.1016/j.jbiotec.2008.07.1045.
Andersen RA. 2005. Algal Culturing Techniques. Elsevier Inc.
APHA-AWWA. 1992. Water Environment Federation. Standard methods for the examination of water and wastewater (18 th ed.). Washington, DC.
Aslanbay Guler B, Deniz I, Demirel Z, Oncel SS, Imamoglu E. 2019. Comparison of different photobioreactor configurations and empirical computational fluid dynamics simulation for fucoxanthin production. Algal Research, 37, 195-204. https://doi.org/https://doi.org/10.1016/j.algal.2018.11.019.
Bland E, Angenent LT. 2016. Pigment-targeted light wavelength and intensity promotes efficient photoautotrophic growth of Cyanobacteria. Bioresource Technology, 216, 579-586. https://doi.org/http://dx.doi.org/10.1016/j.biortech.2016.05.116.
Boussiba S, Richmond A. 1979. Isolation and purification of phycocyanins from the blue-green alga Spirulina platensis. Archive of Microbiology, 120, 155-159.
Chen CY, Kao PC, Tsai CJ, Lee DJ, Chang JS. 2013. Engineering strategies for simultaneous enhancement of C-phycocyanin production and CO2 fixation with Spirulina platensis. Bioresource Technology, 145, 307-312. https://doi.org/http://dx.doi.org/10.1016/j.biortech.2013.01.054.
Craggs R, Park J, Heubeck S, Sutherland D. 2014. High rate algal pond systems for low-energy wastewater treatment, nutrient recovery and energy production. New Zealand Journal of Botany, 52(1), 60-73. https://doi.org/10.1080/0028825X.2013.861855.
de Morais M, Vieira Costa J. 2007. Biofixation of carbon dioxide by Spirulina sp. and Scenedesmus obliquus cultivated in a three-stage serial tubular photobioreactor. Journal of Biotechnology, 129, 439-445. https://doi.org/10.1016/j.jbiotec.2007.01.009.
Dejsungkranont M, Chen HH, Sirisansaneeyakul S. 2017. Enhancement of antioxidant activity of C-phycocyanin of Spirulina powder treated with supercritical fluid carbon dioxide. Agriculture and Natural Resources, 51(5), 347-354. https://doi.org/https://doi.org/10.1016/j.anres.2017.12.001.
Draaisma RB, Wijffels RH, Slegers PM, Brentner LB, Roy A, Barbosa MJ. 2013. Food commodities from microalgae. Current Opinion in Biotechnology, 24(2), 169-177. https://doi.org/https://doi.org/10.1016/j.copbio.2012.09.012.
Ghorbani A, Rahimpour HR, Ghasemi Y, Zoughi S, Rahimpour MR. 2014. A Review of Carbon Capture and Sequestration in Iran: Microalgal Biofixation Potential in Iran. Renewable and Sustainable Energy Reviews, 35, 73-100. https://doi.org/http://dx.doi.org/10.1016/j.rser.2014.03.013.
Gonçalves AL, Rodrigues CM, Pires JCM, Simões M. 2016. The effect of increasing CO2 concentrations on its capture, biomass production and wastewater bioremediation by microalgae and cyanobacteria. Algal Research, 14, 127-136. https://doi.org/http://dx.doi.org/10.1016/j.algal.2016.01.008.
González López CV, García MdCC, Fernández FGA, Bustos CS, Chisti Y, Sevilla JMF. 2010. Protein measurements of microalgal and cyanobacterial biomass. Bioresource Technology, 101(19), 7587-7591. https://doi.org/https://doi.org/10.1016/j.biortech.2010.04.077.
Gordillo F, Jimenez C, Figueroa F, Niell F. 1999. Effects of increased atmospheric CO2 and N supply on photosynthesis, growth and cell composition of the cyanobacterium Spirulina platensis (Arthrospira). Journal of Applied Phycology, 10, 461-469.
Gordillo FJL, Jiménez C, Goutx M, Niell X. 2001. Effects of CO2 and nitrogen supply on the biochemical composition of Ulva rigida with especial emphasis on lipid class analysis. Journal of Plant Physiology, 158(3), 367-373. https://doi.org/http://dx.doi.org/10.1078/0176-1617-00209.
Ho SH, Lu WB, Chang JS. 2012. Photobioreactor strategies for improving the CO2 fixation efficiency of indigenous Scenedesmus obliquus CNW-N: Statistical optimization of CO2 feeding, illumination, and operation mode. Bioresource Technology, 105, 106-113. https://doi.org/http://dx.doi.org/10.1016/j.biortech.2011.11.091.
Jacob-Lopes E, Cacia Ferreira Lacerda LM, Franco TT. 2008. Biomass production and carbon dioxide fixation by Aphanothece microscopica Nägeli in a bubble column photobioreactor. Biochemical Engineering Journal, 40(1), 27-34. https://doi.org/http://dx.doi.org/10.1016/j.bej.2007.11.013.
Jacob-Lopes E, Scoparo CHG, Franco TT. 2008. Rates of CO2 removal by Aphanothece microscopica Nägeli in tubular photobioreactors. Chemical Engineering and Processing: Process Intensification, 47(8), 1365-1373. https://doi.org/http://dx.doi.org/10.1016/j.cep.2007.06.004.
Johnson T, Katuwal S, Anderson G, Gu L, Zhou R, Gibbons W. 2018. Photobioreactor Cultivation Strategies for Microalgae and Cyanobacteria. Biotechnology Progress, 34. https://doi.org/10.1002/btpr.2628.
Koller M, Muhr A, Braunegg G. 2014. Microalgae as versatile cellular factories for valued products. Algal Research, 6, 52-63. https://doi.org/https://doi.org/10.1016/j.algal.2014.09.002.
Lowry OH, Rosebrough JN, Farr A, Randall R. 1951. Protein measurement with the Folin Phenol Reagent. The Journal of Biological Chemistry, 193, 265-275.
Mary Leema JT, Kirubagaran R, Vinithkumar NV, Dheenan PS, Karthikayulu S. 2010. High value pigment production from Arthrospira (Spirulina) platensis cultured in seawater. Bioresource Technology, 101(23), 9221-9227. https://doi.org/http://dx.doi.org/10.1016/j.biortech.2010.06.120.
Michael A, Kyewalyanga MS, Lugomela CV. 2019. Biomass and nutritive value of Spirulina (Arthrospira fusiformis) cultivated in a cost-effective medium. Annals of Microbiology, 69(13), 1387-1395. https://doi.org/10.1007/s13213-019-01520-4.
Mohsenpour SF, Willoughby N. 2016. Effect of CO2 aeration on cultivation of microalgae in luminescent photobioreactors. Biomass and Bioenergy, 85, 168-177. https://doi.org/http://dx.doi.org/10.1016/j.biombioe.2015.12.002.
Morais MGd, Costa ALH. 2007. Biofixation of carbon dioxide by Spirulina sp. and Scenedesmus obliquus cultivated in a three-stage serial tubular photobioreactor. Journal of Biotechnology, 129, 439-445. https://doi.org/10.1016/j.jbiotec.2007.01.009.
Ores JdC, Amarante MCAd, Kalil SJ. 2016. Co-production of carbonic anhydrase and phycobiliproteins by Spirulina sp. and Synechococcus nidulans. Bioresource Technology, 219, 219-227. https://doi.org/http://dx.doi.org/10.1016/j.biortech.2016.07.133.
Rangel-Yagui Cde O, Danesi ED, de Carvalho JC, Sato S. 2004. Chlorophyll production from Spirulina platensis: cultivation with urea addition by fed-batch process. Bioresource Technology, 92(2), 133-141. https://doi.org/10.1016/j.biortech.2003.09.002.
Vanthoor-Koopmans M, Wijffels RH, Barbosa MJ, Eppink MHM. 2013. Biorefinery of microalgae for food and fuel. Bioresource Technology, 135, 142-149. https://doi.org/https://doi.org/10.1016/j.biortech.2012.10.135.
Whitton R, Ometto F, Pidou M, Jarvis P, Villa R, Jefferson B. 2015. Microalgae for municipal wastewater nutrient remediation: mechanisms, reactors and outlook for tertiary treatment. Environmental Technology Reviews, 4(1), 133-148. https://doi.org/10.1080/21622515.2015.1105308.
Zhao B, Su Y, Zhang Y, Cui G. 2015. Carbon dioxide fixation and biomass production from combustion flue gas using energy microalgae. Energy, 89, 347-357. https://doi.org/http://dx.doi.org/10.1016/j.energy.2015.05.123.
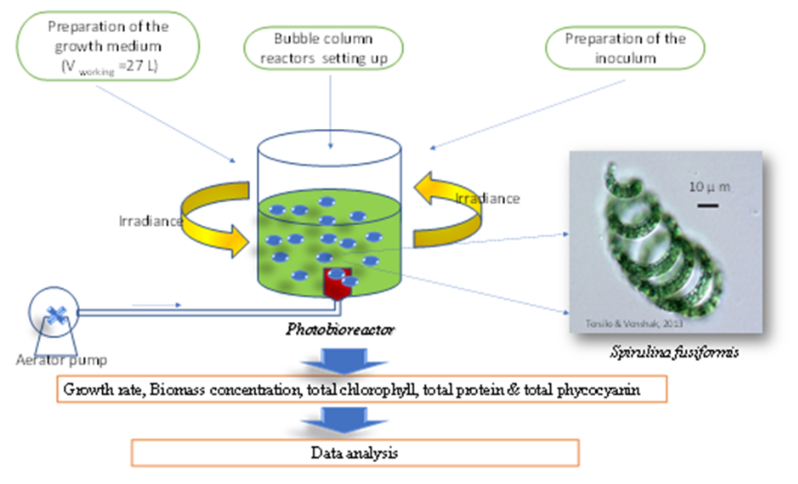